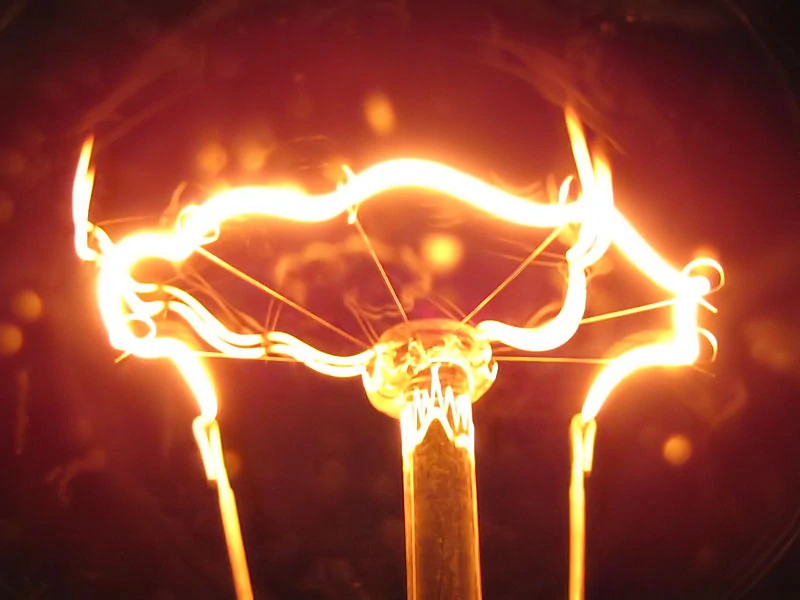
Light
Light is a phenomenon that occurs through the pulsing of energy, which propagates through space. This energy has a frequency that the human eye can perceive as light.
It is important to note that the human eye doesn't see the light itself, but only the atoms illuminated by that light.
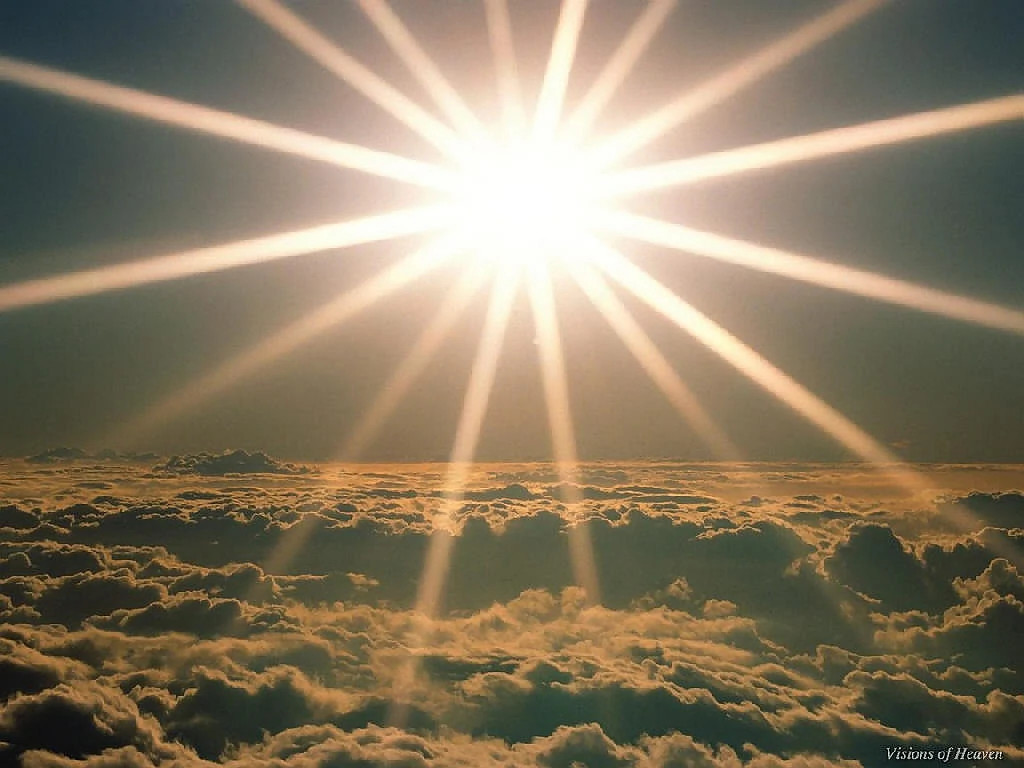
Light is a key phenomenon that allows us to study the properties of energy. It is merely the visible part of pulsing energy (in modern physics, this is referred to as the visible portion of the electromagnetic wave spectrum).
For centuries, light was the only means to study distant phenomena in the universe. Most of our knowledge about the cosmos comes from this method of observation.
It was only in the 20th century, when we began to utilize other parts of the spectrum, such as radio waves and X-rays, that we gained new insights into how nature and the entire universe are structured.
For example, the study of X-rays from space confirmed that energy can concentrate in space even without the presence of matter, supporting Einstein's famous equation m = e⋅ c2. This validates what had already been derived through technical and mathematical methods in the 20th century.
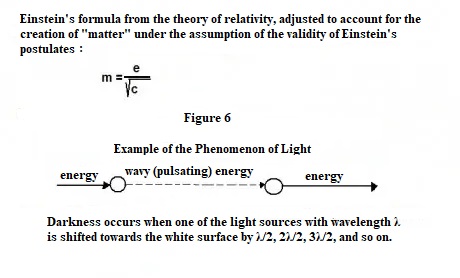
Using telescopes, we can indirectly observe phenomena such as gravity, mass, and other cosmic events that arise from the nature and properties of energy. You will learn more about these phenomena in subsequent chapters of Reciprocal Physics.
However, we can directly observe the behavior and principles of energy because light is a pulsing form of energy whose frequency does not change its fundamental properties. This entire chapter is dedicated to exploring this phenomenon.
Note: In current physical hypotheses, there is no unified view on the nature of light. Sometimes it behaves like an electromagnetic wave, while at other times it exhibits properties typical of matter. This inconsistent approach, which separates matter from energy, is unacceptable from a technical standpoint. From Huygens' wave theory to Newton's emission theory, electromagnetic theory, and quantum theory—each of these approaches has its shortcomings.
However, none of these theories can satisfactorily explain all emerging and existing phenomena. Moreover, systematic analysis reveals numerous contradictions between these theories and objective natural phenomena and principles. Reciprocal Physics, which is based on the validity of Einstein's theses, is unable to effectively utilize current scientific hypotheses about the nature of light. When applying these hypotheses, contradictions with the problem's premise—namely, objective natural laws—arise after only a few steps.
Due to its properties, light allows us to examine and understand various laws and functions of energy without needing to observe the emergence of any force. For thousands of years, it has also helped us uncover natural laws that would otherwise remain hidden.
In Figure 6, you can see how light is created. Non-pulsating energy passes through a pulsation source, such as a light bulb. Pulsating atoms in the filament of the bulb transfer their motion to the energy, which then propagates further as pulsating light. Similarly, other frequencies are generated that we cannot see with the naked eye but can be detected using instruments.
When pulsating energy encounters something that does not transmit the pulsation, such as the retina of the eye, it begins to vibrate it. The eye perceives the light, and the energy pulses adjust to match the retina.
The energy then continues through the head as a non-pulsating frequency that the eye perceives, while maintaining its direction.
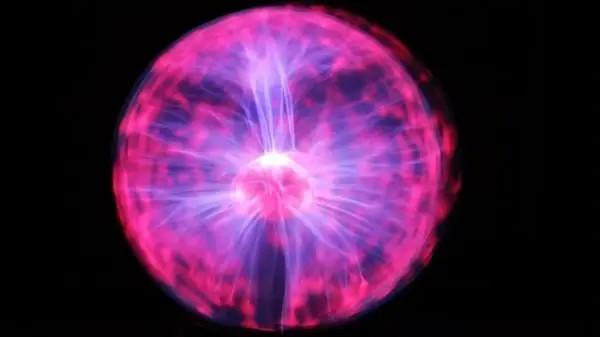
Energy pulses can be canceled out in ways other than just by hitting an object. An interesting effect is the creation of darkness from two glowing lights. How does this work? If we place two light sources at equal distances from a white surface in a dark room, their beams combine and the light intensity doubles.
However, if one of the sources is moved closer to the white surface (as shown in Figure 6), darkness is created. In this case, the light pulses cancel each other out and produce a non-pulsating beam that no longer creates visible light.
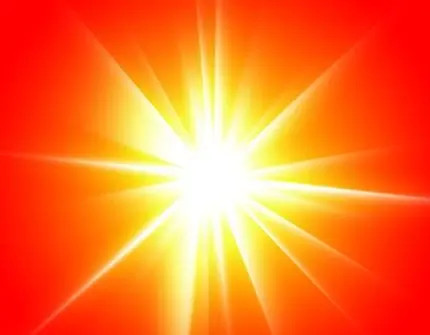
Energy spreads out in all directions and in every direction.
We can easily verify this through light. When we look at the night sky, we see light coming from every direction. Similarly, light from a light bulb spreads out in all directions around it.
Energy can move at various speeds.
The speed of light (pulsating energy) changes depending on the medium through which it passes. For example, light travels differently in a vacuum, in air, and in glass. This fact arises from Einstein's formula E=mc^2, as shown in Figure 3, Example 7a.
Similar to sound, light also exhibits the Doppler effect. This effect shows that the wavelength and frequency of light change depending on the relative speed between the light source and the observer.
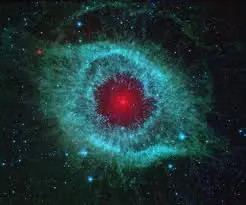
Around bodies (both massive and solid), energy becomes concentrated.
We can easily verify this fact using light and our own eyes.
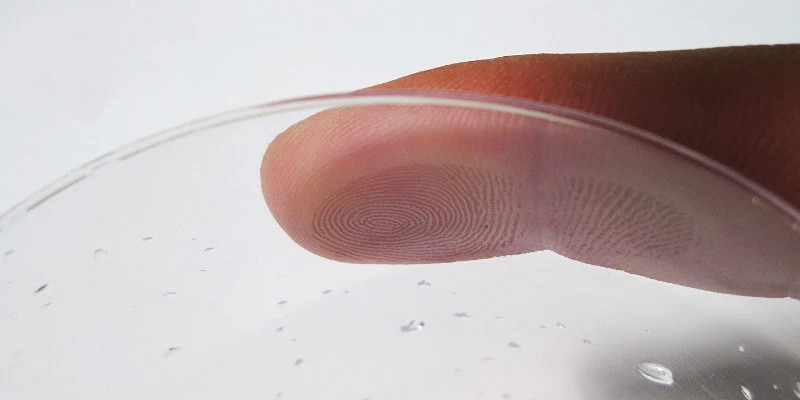
When we look at a glass prism, we see that it reflects light from two different surfaces. If we gently press these surfaces with our fingers (preferably moistened), the light reflection at the point of contact disappears. Once we remove our fingers, the reflection reappears. This indicates that there is no permanent deformation of the glass affecting the reflection or refraction of light. This simple experiment demonstrates how energy is concentrated around bodies and how we can explore it even with the touch of our fingers.
As previously mentioned, when the speed of energy changes, it becomes concentrated according to the formula mc = constant. It is known that light travels more slowly through glass than in a vacuum. The same phenomenon occurs in air or water.
This means that the speed at which energy propagates varies depending on the density of the medium. As mentioned earlier, energy is essentially a form of matter (or matter is a form of energy), so it must concentrate itself before penetrating a material body. Even though glass has only a slightly higher density than a vacuum, there is a visible concentration of energy when light passes through glass.
Since we can easily alter this thin layer with our fingers, for example by pressing on it, it is clear that this layer is not part of the glass itself. This layer of concentrated energy appears to us as shine or reflection of light. If the layer of concentrated energy is absent, the reflection or shine of light does not appear. The strength or intensity of this layer depends on the structure of the material and its distance from similar layers of energy in the surrounding environment.
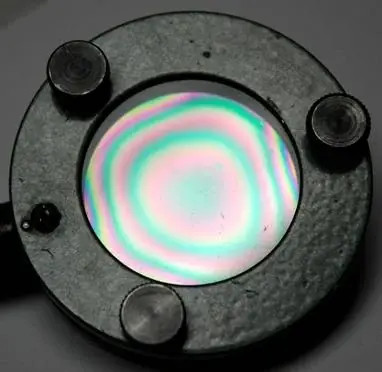
When the layer of concentrated energy around an object is not restricted by anything, the reflection and shine of light are even and compact. This is because the light reflects and spreads uniformly. A different situation occurs if this layer is locally restricted or weakened.
In such situations, irregular reflections and refractions of light occur. These phenomena are best observed with film slides placed between glass plates. Distortions in the film where it touches the glass plate create uneven density distribution in the energy layer. As a result, various irregular reflections and refractions of light are produced. This phenomenon, known as Newton's rings, appears due to these irregularities and is evidence of how small changes in the density of the energy layer can affect the propagation and perception of light.
Note: Currently, there are several different opinions on the origin of Newton's rings. Some publications claim that this phenomenon is not fully understood, while others suggest that it is caused by the presence of air. However, these explanations are unsatisfactory because Newton's rings also appear in a vacuum, where air is not present. This suggests that their formation is not dependent on the presence of air but rather on other factors.
The layer of concentrated energy on the surface of bodies is a key cause of the phenomenon known as light refraction. The effects of this phenomenon are visible in Figure 7, Examples 7a and 7b. Light refraction is the result of several factors that combine to produce the overall effect.
Firstly, a certain frequency of energy pulses, which are perceived by the human eye, passes through a material body, such as glass or water.
Secondly, as mentioned, the direction of movement of individual energy rays bends. This bending is caused by the thinning of energy around large bodies in the universe (for example, the curvature of space around massive objects).
In the case of light refraction, however, the bending of direction is opposite—energy is deflected away from the body due to the concentrated layer. The extent of this deflection depends on the strength and size of the layer. This layer not only refracts the spreading light beam but is also influenced by the same frequency, contributing to the overall effect of light refraction.
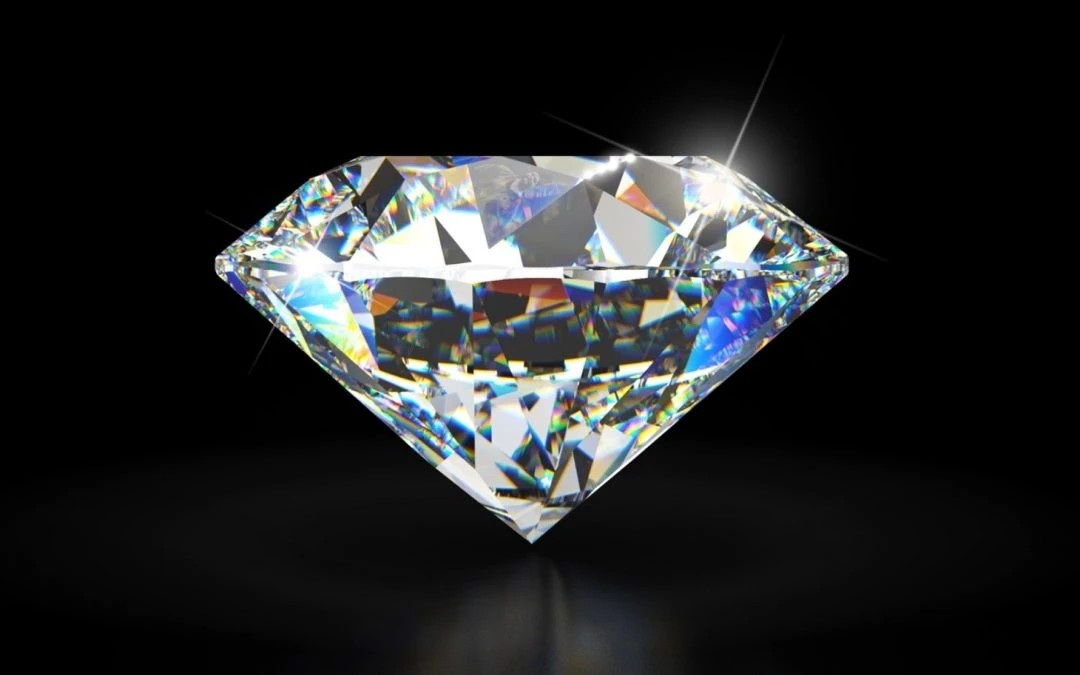
At the edge of material bodies, the speed of energy, including the speed of light, slows down, leading to the formation of a layer of concentrated energy. When light passes through a body that is denser than the surrounding medium (such as glass, diamond, or water), it slows down and creates this concentrated layer around the body. Once the light exits the body, it speeds up back to its original speed. If the light is pulsed (which is typical for pulsating energy), it causes the layer of concentrated energy at the edge of the body to also pulse. Just as in the retina of the eye, where light pulses are canceled out, the light continues to spread as non-pulsating energy. When the layer of concentrated energy is absent at the interface of two different densities, there is no reflection of light.
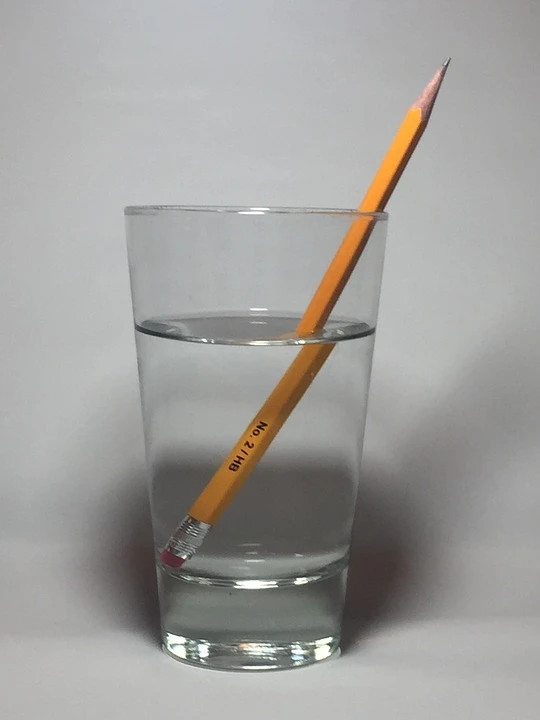
In Figure 7, Example 7a, the effect known as light refraction is illustrated. A light ray striking the edge of a glass plate encounters a layer of concentrated energy and causes it to vibrate. Different colors of light have different frequencies; for example, red and violet bend in different directions. As the ray exits the glass layer, the speed of light increases back to its original speed. Due to the layer of concentrated energy, the directions of the rays align, and both colors continue traveling parallel to each other. In Figure 7, Example 7b, the layers of concentrated energy are oriented differently, causing a greater dispersion of colors and creating a visible color spectrum.
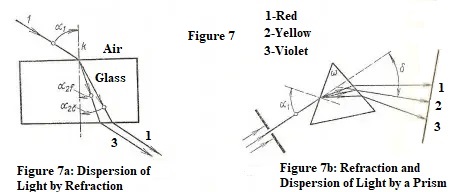
In Figure 7, Example 7a, the reflection of light is depicted. When a light ray strikes the edge of a glass plate, it enters the layer of concentrated energy. If the plate is transparent, part of the light that passes through this layer vibrates and continues through the glass.
The second part of the light that hits the layer of concentrated energy is reflected back from the plate. When the plate is opaque, such as a mirror, the entire light ray vibrates and undergoes total reflection. In this case, the angle of incidence of the light is equal to the angle of reflection.
By combining different crystalline structures and orientations in a transparent plate, we can create various optical effects. For example, with birefringent calcite, which has a specific structure, double refraction occurs, producing intriguing and complex optical patterns.
The transparency of materials depends on their surface and internal structure.
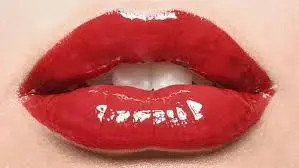
Glossy surfaces are those where the surface irregularities are smaller than the height of the layer of concentrated energy on their surface. The shine we see is actually the reflection of light from this layer.
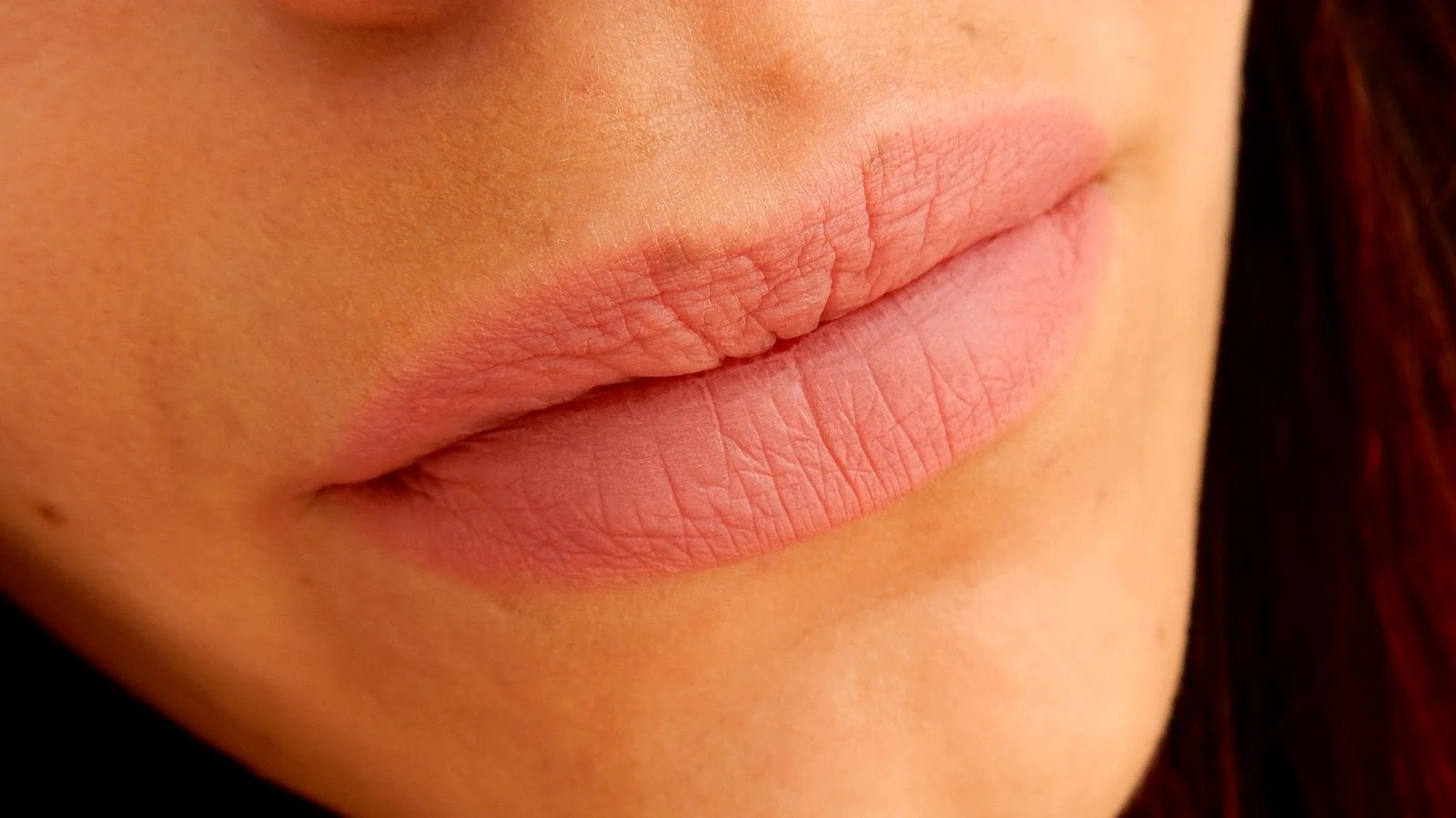
Matte surfaces have surface irregularities larger than the height of the layer of concentrated energy, which prevents the reflection of light and causes it to scatter. This scattering gives the surface a matte appearance.
Matte surfaces can still transmit various frequencies of energy, such as X-rays or radio waves, which are not detectable by our eyes.
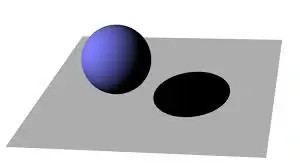
Opaque materials are substances with internal structures that prevent the passage of pulsating energy, such as light. These materials block or absorb energy, including frequencies that the human eye cannot see, thereby preventing it from passing through.
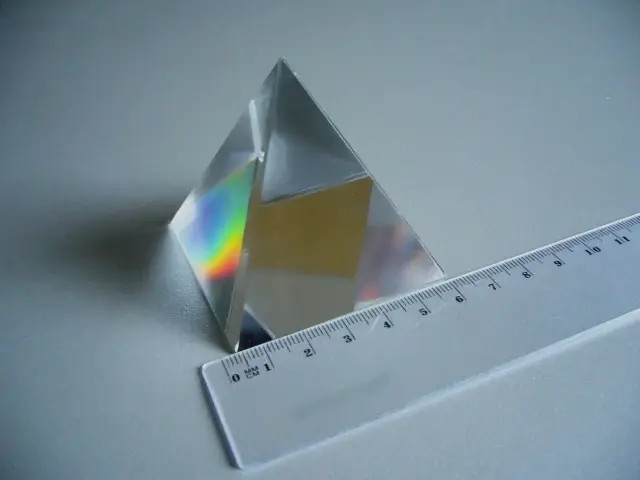
When we look at a glass plate or prism, we often find that ideal transparency is not perfect. Instead, small leaks of light may be visible. These leaks are caused by imperfections in the material's structure, the bending of light around atoms, and other factors.
The dispersion of light, as shown in Figure 3, Example 7a, results from a combination of light bending and reflection. The strength of the layer of concentrated energy affects how much the light bends. For instance, a diamond has a stronger layer of concentrated energy than water, meaning that light bends more in a diamond.
All phenomena related to light, including refraction and dispersion, can be explained by interactions between energy and matter in different states. Essentially, there are no additional mysteries—everything we see is simply the result of these straightforward interactions and the concentration of energy.
For more detailed information about light, you can refer to the publication titled Reciprocal Physics, which is available for download in the download section.